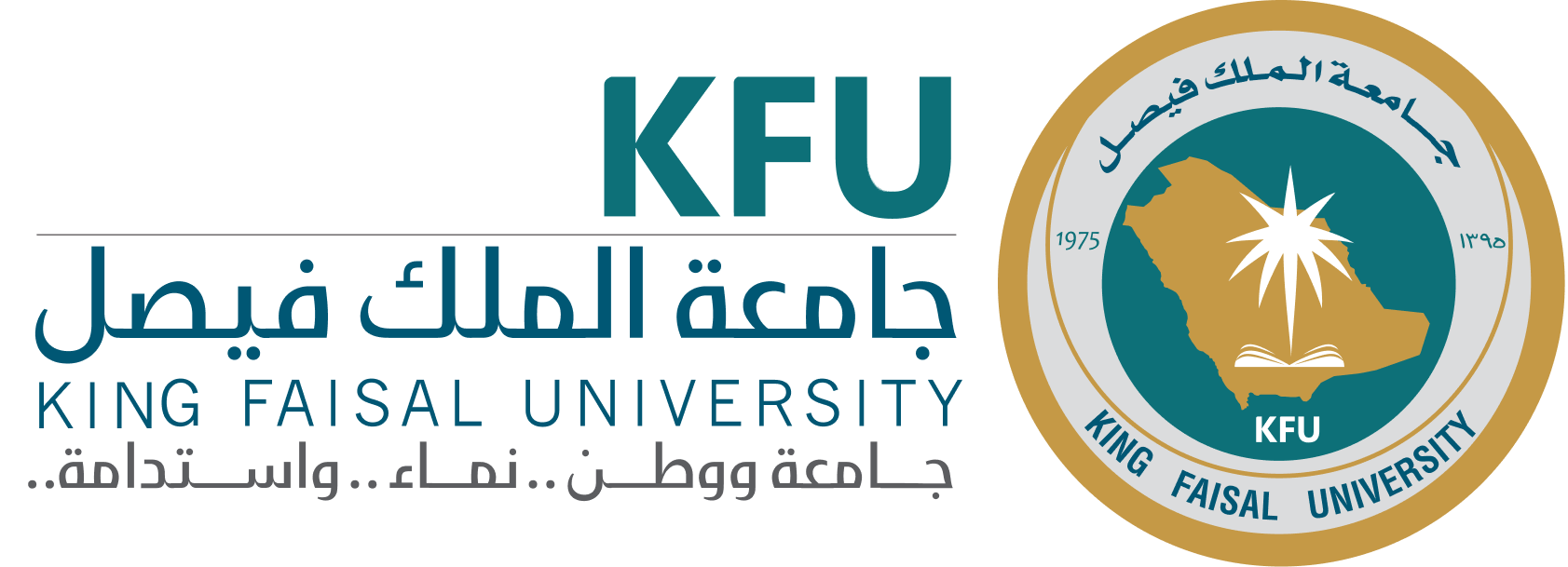
Scientific Journal Of King Faisal University: Basic and Applied Sciences
Scientific Journal of King Faisal University: Basic and Applied Science
Performance Optimisation of a Wind Turbine Simulator with Transverse Cracked Blades using Taguchi-Based Grey Relational Analysis
(Abdulhamed Hamdan Al-Hinai, Karu Clement Varaprasad and V. Vinod Kumar)Abstract
The presence of blade surface cracks creates chances for vibration propagation that affects wind turbine performance. This study examines the effects of transverse cracks on the vibration levels and power output of wind turbine systems. Multiple regression models are developed as predictive tools to highlight the impact of transverse crack sizes and variations in rotational speed on vibration levels and power generation. Grey relational analysis is used as an optimisation technique to identify optimal settings for maximum power output and minimum vibration levels. By modelling and analysing these factors, the study reveals a significant correlation between increased crack size and elevated vibration levels, which can compromise structural integrity. Additionally, while higher rotational speeds initially boost power output, they also lead to exponential increases in vibration, exacerbating the risks associated with blade defects. The findings emphasise the critical need for a balanced approach in optimising turbine performance, introducing a novel approach to speed management and vibration control, which is employed in this study. The research suggests potential avenues for future exploration, including the development of advanced materials and design innovations aimed at mitigating these risks, enabling safer and more efficient turbine operation.
KEYWORDS
Defect, optimal settings, power, rotational speed, regression, vibration
PDF
References
Al-Hinai, A., Varaprasad, K.C. and Kumar, V.V. (2024, June). Analytical Simulation Approach to Evaluate the Ambient Humidity Effects on the Performance of a Wind Accelerator. In IOP Conference Series: Earth and Environmental Science, 1365(1), 012006. DOI: 10.1088/1755-1315/1365/1/012006
Balasubramanian, K., Thanikanti, S.B., Subramaniam, U., Sudhakar, N. and Sichilalu, S. (2020). A novel review on optimization techniques used in wind farm modelling. Renewable Energy Focus, 35(n/a), 84–96. DOI: 10.1016/j.ref.2020.09.001
Barszcz, T. (2019). Standard Vibration Analysis Methods. In: T. Barszcz (eds.) Vibration-Based Condition Monitoring of Wind Turbines. Applied Condition Monitoring, Switzerland, Springer Nature Springer, DOI:10.1007/978-3-030-05971-2_2
Blaabjerg, F. and Ma, K. (2017). Wind energy systems. IEEE, 105(11), 2116–31. DOI: 10.1109/JPROC.2017.2695485
Castorrini, A., Greco, E. and Sicilian, M. (2019). Computational analysis of performance deterioration of a wind turbine blade strip subjected to environmental erosion. Computational Mechanics, 64(n/a), 1133–53. DOI: 10.1007/s00466-019-01697-0
Derakhshan, S., Tavaziani, A. and Kasaeian, N. (2015). Numerical shape optimization of a wind turbine blades using artificial bee colony algorithm. Journal of Energy Resources Technology, 137(5), 051210. DOI: 10.1115/1.4031043
Dong, X., Lian, J., Wang, H., Yu, T. and Zhao, Y. (2018). Structural vibration monitoring and operational modal analysis of offshore wind turbine structure. Ocean Engineering, 150(n/a), 280–97. DOI: 10.1016/J.OCEANENG.2017.12.052
Flah, M., Nunez, I., Ben Chaabene, W. and Nehdi, M.L. (2021). Machine learning algorithms in civil structural health monitoring: A systematic review. Archives of Computational Methods in Engineering, 28(4), 2621–43. DOI: 10.1007/s11831-020-09471-9
Florian, M. and Dalsgaard Sørensen, J. (2015). Wind turbine blade life-time assessment model for preventive planning of operation and maintenance. Journal of Marine Science and Engineering, 3(3), 1027–40. DOI: 10.3390/JMSE3031027
He, G., Ding, K., Li, W. and Jiao, X. (2016). A novel order tracking method for wind turbine planetary gearbox vibration analysis based on discrete spectrum correction technique. Renewable Energy, 87(n/a), 364–75. DOI: 10.1016/J.RENENE.2015.10.036
Joshuva, A. and Sugumaran, V. (2017). A data driven approach for condition monitoring of wind turbine blade using vibration signals through best-first tree algorithm and functional trees algorithm: A comparative study. ISA transactions, 67(n/a), 160–72. DOI: 10.1016/j.isatra.2017.02.002
Koulocheris, D., Gyparakis, G., Stathis, A. and Costopoulos, T. (2013). Vibration signals and condition monitoring for wind turbines. Engineering, 5(12), 948. DOI: 10.4236/eng.2013.512116
Kusnick, J., Adams, D.E. and Griffith, D.T. (2015). Wind turbine rotor imbalance detection using nacelle and blade measurements. Wind Energy, 18(2), 267–76. DOI: 10.1002/we.1696
Liu, X., Lu, C., Liang, S., Godbole, A. and Chen, Y. (2015). Influence of the vibration of large-scale wind turbine blade on the aerodynamic load. Energy Procedia, 75(n/a), 873–9. DOI: 10.1016/j.egypro.2015.07.196
Liu, Y., Cheng, H., Kong, X., Wang, Q. and Cui, H. (2019). Intelligent wind turbine blade icing detection using supervisory control and data acquisition data and ensemble deep learning. Energy Science & Engineering, 7(6), 2633–45. DOI: 10.1002/ese3.449
Liu, Z., Wang, X. and Zhang, L. (2020). Fault diagnosis of industrial wind turbine blade bearing using acoustic emission analysis. IEEE Transactions on Instrumentation and Measurement, 69(9), 6630–9. DOI: 10.1109/TIM.2020.2969062
Lubosny, Z. (2003). Wind Turbine Generator Systems. In: Z. Lubosny (eds.) Wind Turbine Operation in Electric Power Systems. Power Systems, Berlin, Germany, Springer. DOI: 10.1007/978-3-662-10944-1_2
Maheswari, R.U. and Umamaheswari, R. (2017). Trends in non-stationary signal processing techniques applied to vibration analysis of wind turbine drive train–A contemporary survey. Mechanical Systems and Signal Processing, 85(n/a), 296–311. DOI: 10.1016/J.YMSSP.2016.07.046
Ou, Y., Chatzi, E.N., Dertimanis, V.K. and Spiridonakos, M.D. (2017). Vibration-based experimental damage detection of a small-scale wind turbine blade. Structural Health Monitoring, 16(1), 79–96. DOI: 10.1177/1475921716663876
Pacheco, J., Pimenta, F., Guimarães, S., Castro, G., Cunha, Á., Matos, J.C. and Magalhães, F. (2024). Experimental evaluation of fatigue in wind turbine blades with wake effects. Engineering Structures, 300(n/a), 117140. DOI: 10.1016/j.engstruct.2023.117140
Polinder, H. (2011). Overview of and trends in wind turbine generator systems. In: IEEE Power and Energy Society General Meeting, Detroit, MI, USA, 24–28/07/2011. DOI: 10.1109/PES.2011.6039342
Riddle, T.W., Nelson, J.W. and Cairns, D.S. (2018). Effects of defects in composite wind turbine blades–Part 3: A framework for treating defects as uncertainty variables for blade analysis. Wind Energy Science, 3(1), 107–20. DOI: 10.5194/wes-3-107-2018
Sarkar, S. and Chakraborty, A. (2018). Optimal design of semiactive MR‐TLCD for along‐wind vibration control of horizontal axis wind turbine tower. Structural Control and Health Monitoring, 25(2), e2083. DOI: 10.1002/stc.2083
Sheng, S. (2012). Wind turbine gearbox condition monitoring round robin study-vibration analysis (No. NREL/TP-5000-54530). National Renewable Energy Lab. (NREL), Golden, CO (United States). DOI: 10.2172/1048981
Skrimpas, G.A., Kleani, K., Mijatovic, N., Sweeney, C.W., Jensen, B.B. and Holboell, J. (2016). Detection of icing on wind turbine blades by means of vibration and power curve analysis. Wind Energy, 19(10), 1819–32. DOI: 10.2172/9460
Tchakoua, P., Wamkeue, R., Ouhrouche, M., Slaoui-Hasnaoui, F., Tameghe, T.A. and Ekemb, G. (2014). Wind turbine condition monitoring: State-of-the-art review, new trends, and future challenges. Energies, 7(4), 2595–630. DOI: 10.3390/EN7042595
Teng, W., Ding, X., Cheng, H., Han, C., Liu, Y. and Mu, H. (2019). Compound faults diagnosis and analysis for a wind turbine gearbox via a novel vibration model and empirical wavelet transform. Renewable Energy, 136(n/a), 393–402. DOI: 10.1016/j.renene.2018.12.094
Tibaldi, C., Kim, T., Larsen, T.J., Rasmussen, F., Rocca Serra, R.D. and Sanz, F. (2016). An investigation on wind turbine resonant vibrations. Wind energy, 19(5), 847–59. DOI: 10.1002/WE.1869
Wagner, H. (2015). Introduction to wind energy systems. EPJ Web of Conferences, 98(n/a), n/a. DOI: 10.1051/epjconf/20159804002
Wang, J., Zhang, L., Huang, X., Zhang, J. and Yuan, C. (2022). Initiation mechanism of transverse cracks in wind turbine blade trailing edge. Energy Eng, 119(1), 407–18. DOI: 10.32604/ee.2022.016439
Xie, F. and Aly, A.M. (2020). Structural control and vibration issues in wind turbines: A review. Engineering Structures, 210(n/a), 110087. DOI: 10.1016/j.engstruct.2019.110087
Yang, W., Lang, Z. and Tian, W. (2015). Condition monitoring and damage location of wind turbine blades by frequency response transmissibility analysis. IEEE Transactions on Industrial Electronics, 62(10), 6558–64. DOI: 10.1109/TIE.2015.2418738
Yang, W., Tavner, P.J., Crabtree, C.J. and Wilkinson, M. (2009). Cost-effective condition monitoring for wind turbines. IEEE Transactions on Industrial Electronics, 57(1), 263–71. DOI: 10.1109/TIE.2009.2032202